Location: 41.77970N 12.24816E
In the following sections, we will concentrate on the overall coastal sedimentation and erosion processes, on the structural aspects of the breakwaters and on the port capacity. Our aim is to understand how it happened and how it was built.
General layout of Portus Claudius
A few words on coastal morphodynamics
Hypothetical sequence of breakwater construction
Fiumicino Canale – Fossa Traiana
Structural design of the north breakwater
How safe was Portus Claudius?
wave penetration inside Portus Claudius
General layout of Portus Trajanus
Berthing capacity of Portus Trajanus
Pictures of the port
Thousands of documents deal with Portus Claudius, Portus Trajanus or Portus Augusti Ostiensis. The oldest pictures of Portus Claudius are on Nero’s coins of one sestertius (64 AD).
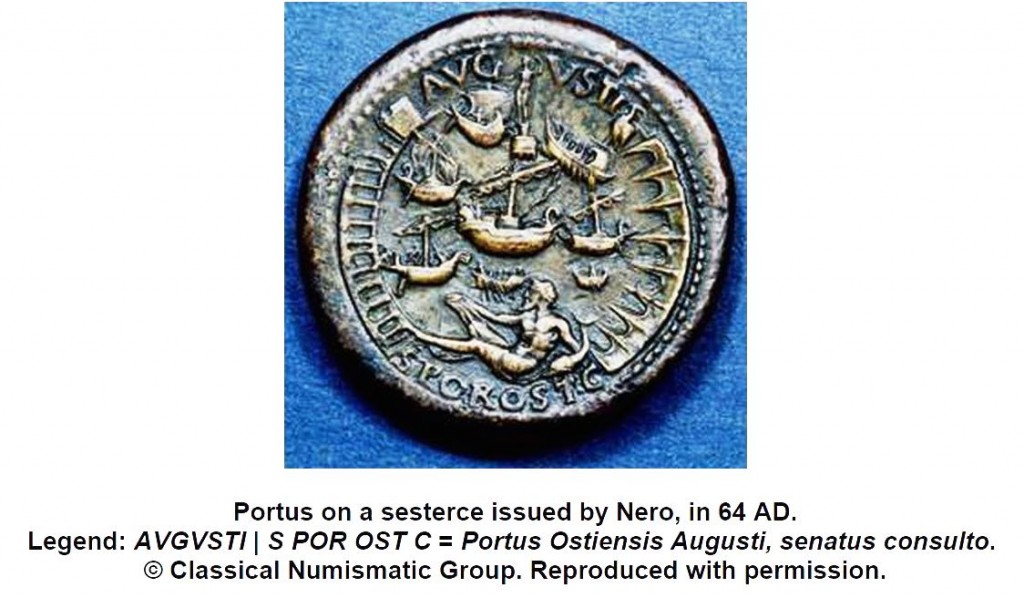
Source: www.ostia-antica.com
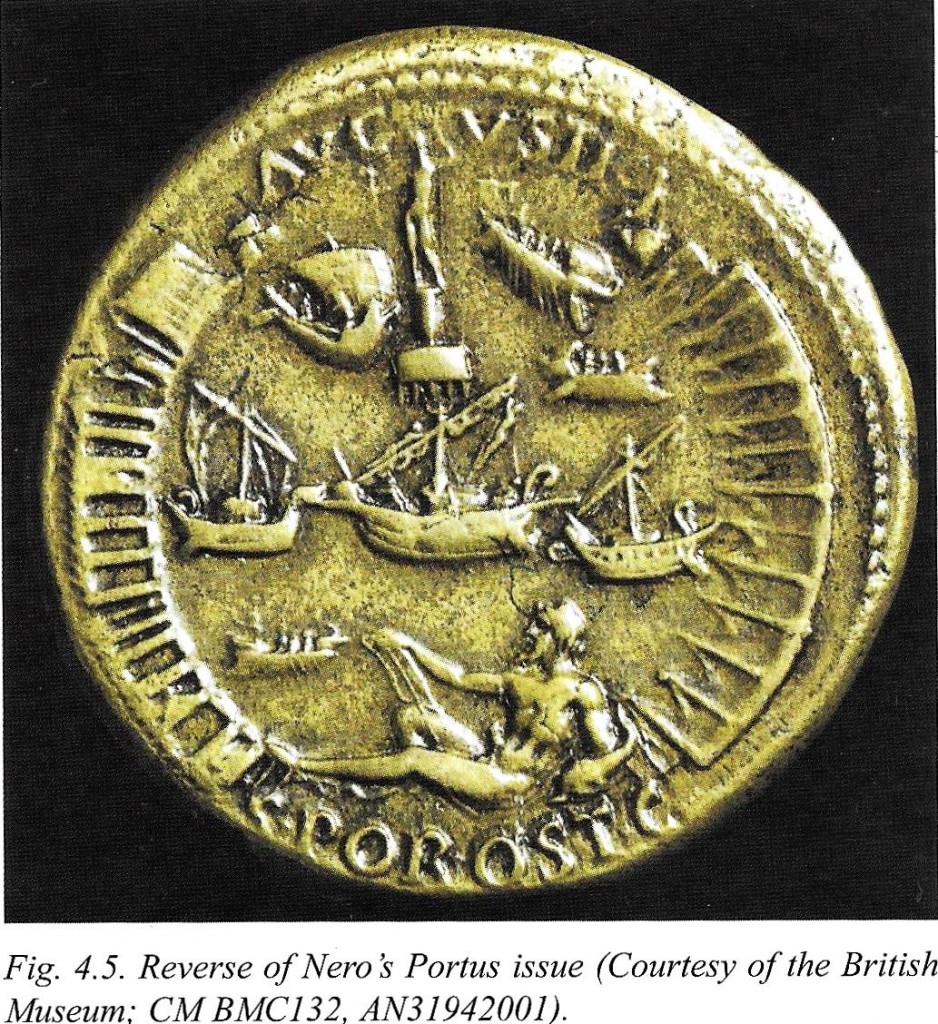
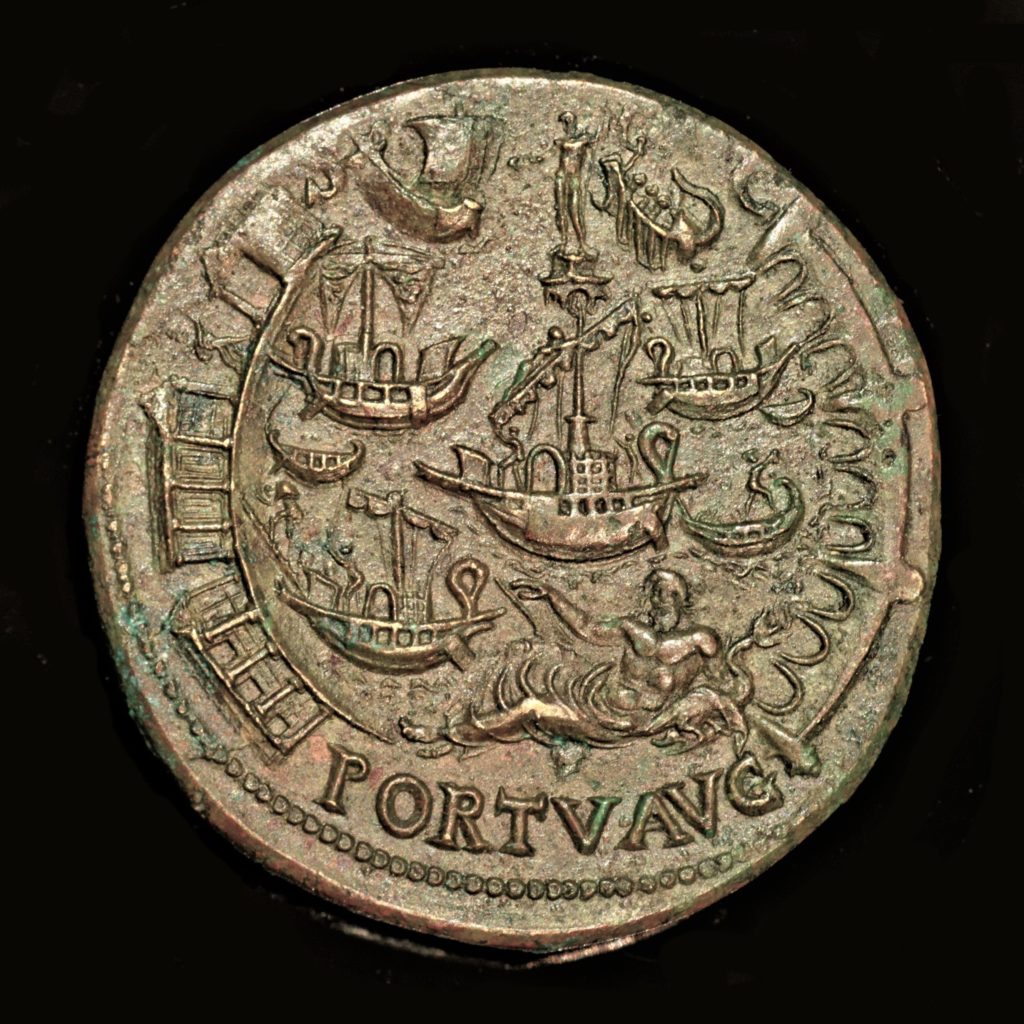
The sea is on top of the pictures, north is to the right.
The right, or north, breakwater has been interpreted (first by Pirro Ligorio in 1554) as an open breakwater supposed to allow water flowing through it.
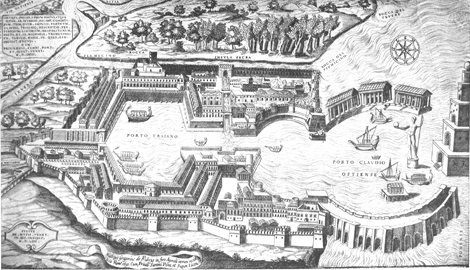
On the British Museum coin, we might even see water flowing around the arch piers, very much like the bow wave of a ship. This concept of ‘arched breakwater‘ was designed to avoid harbour siltation, and similar ‘pilae‘ constructions were found in Puteoli, Misenum and Nisida, in the Bay of Naples, but no ancient literary evidence is available.
The left, or south, breakwater supports a row of buildings (warehouses? or just an artist’s impression of Claudius’ portico?) with a larger structure at the seaward end (temple? lighthouse?).
At the entrance, between both breakwater heads, a large statue seems to represent the well-known lighthouse island.
A ship is leaving the port under oar on the right side and another ship is entering the port under sail on the left side.
Three ships with furled sail are inside the port.
Several smaller boats under oar could be tugs (multiple oars) and service boats (single oar).
For further info see Mary Jane Cuyler (University of Sidney)[22].
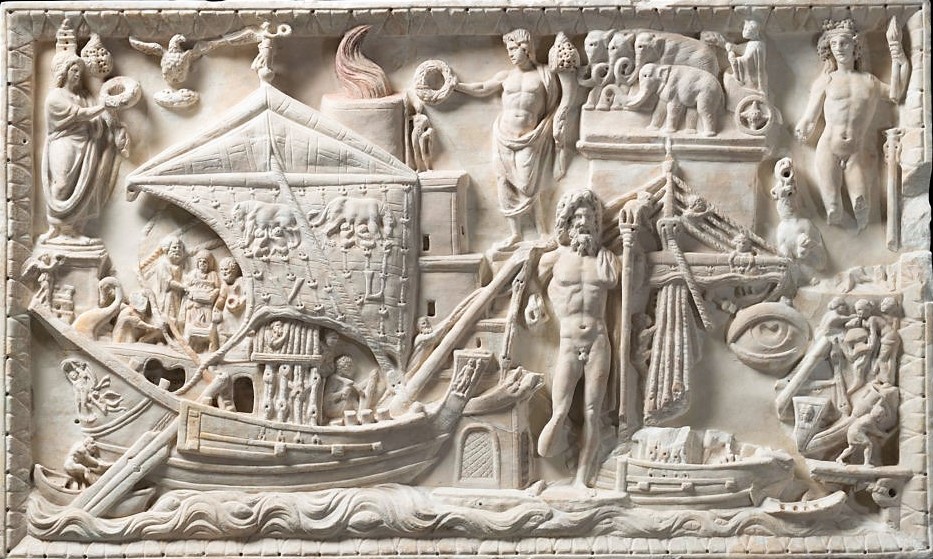
(photo credit: Zètema – Roma Capitale).
For a brilliant description of Portus, see Simon Keay (2014)[28].
A few words on coastal morphodynamics
Coastal engineers are supposed to predict the impact of new coastal structures (i.e. ports, seawalls, man-made beaches, etc.) on the adjacent coastal morphology. Their methodology is usually as follows:
- Understand coastal processes at hand (waves, tides, morphodynamics);
- Build numerical models of these processes (physical scale models are used also) and calibrate them on the past decade(s) if enough data is available;
- Use these models to predict trends over future decade(s).
The following (very) short summary can be deduced from coastal engineering textbooks (e.g., Komar, 1998[18]).
As ports and harbours are supposed to be “low energy” areas (with reduced waves and currents in order to provide sheltering for ships) they are subject to sedimentation.
Sediment (sand and silt) moves both along the coastal zone (longshore littoral drift) and across the coastal zone (cross-shore sediment movement). The coastal zone runs from the dune to a certain water depth (frequently in the order of 10 m). The energy required for sediment motion is mainly provided by wave action (and wind and tidal currents, if any).
- The source of sediment for littoral drift can be fluvial sediment load from river outlets, or erosion of another stretch of the coast. Waves push sediment in front of them when they break with an oblique angle on the coastline. Hence, depending on the wave direction, the rate, and even the direction, of littoral drift can vary in time.
- Cross-shore sediment movement occurs mainly during storms when sediment is taken away from the top of the beach or dune down to deeper water. Reconstruction occurs in milder weather and wind will take fine sediment back to the top of the dune, especially in a tidal area.
Let’s have a look at a typical river outlet with Piero Bellotti[1].
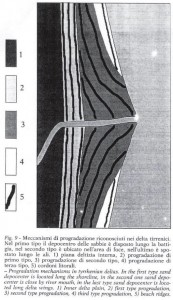
Beach ridges (5) show the progradation of the shoreline due to sediment supply from the river. In this case, wave propagation is perpendicular to the initial shore line (waves move from right to left on this picture). Waves spread the sediment on both sides of the outlet leading to a shape that will remind the Fujiyama (3).
It can be seen also that the total volume of sediment between two equidistant ridges increases in time because the lateral extent is increasing. Hence, the speed of progradation of the outlet cone reduces in time (if the fluvial sediment load is constant).
Obviously, the ratio fluvial sediment load / wave power is a dominant parameter here: more wave action and/or less sediment input lead to a flatter cone, and reverse. If, for some reason, this ratio is reduced (e.g., reduced fluvial sediment load due to reduced fluvial water discharge, due to a drought), the cone will be flattened out and sediment will drift laterally on both sides (4).
What happens if men interact with Nature? e.g., building some obstacle in an area with littoral drift.
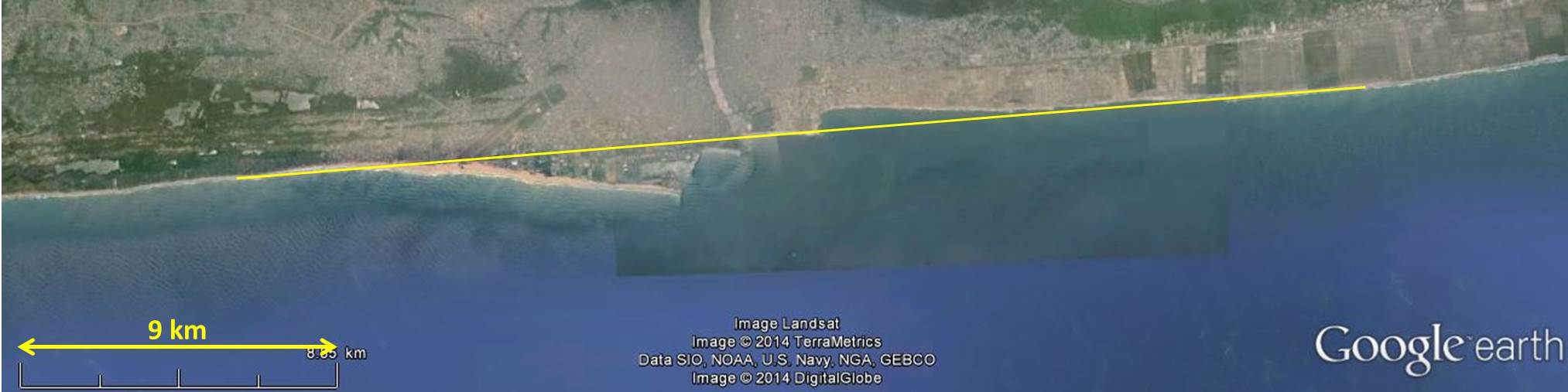
This picture shows the initial shoreline near Cotonou (Benin) (straight yellow line). This was the shoreline before any human construction (a port) was built in the sixties. This coast is known for its littoral drift of around one million cubic meters per year from west to east (left to right on the picture).
Fifty years later, the western shoreline progressed more than 1 km in the offshore direction to the south (i.e. around 20 m/year!). The same volume of sediment was taken away by wave action on the eastern side, inducing erosion over many kilometres … What Nature gives with one hand, she takes back with the other hand.
After some more time, sand will by-pass the harbour entrance which will gradually silt up and reduce draught for navigation[21].
Claudius’ southern breakwater
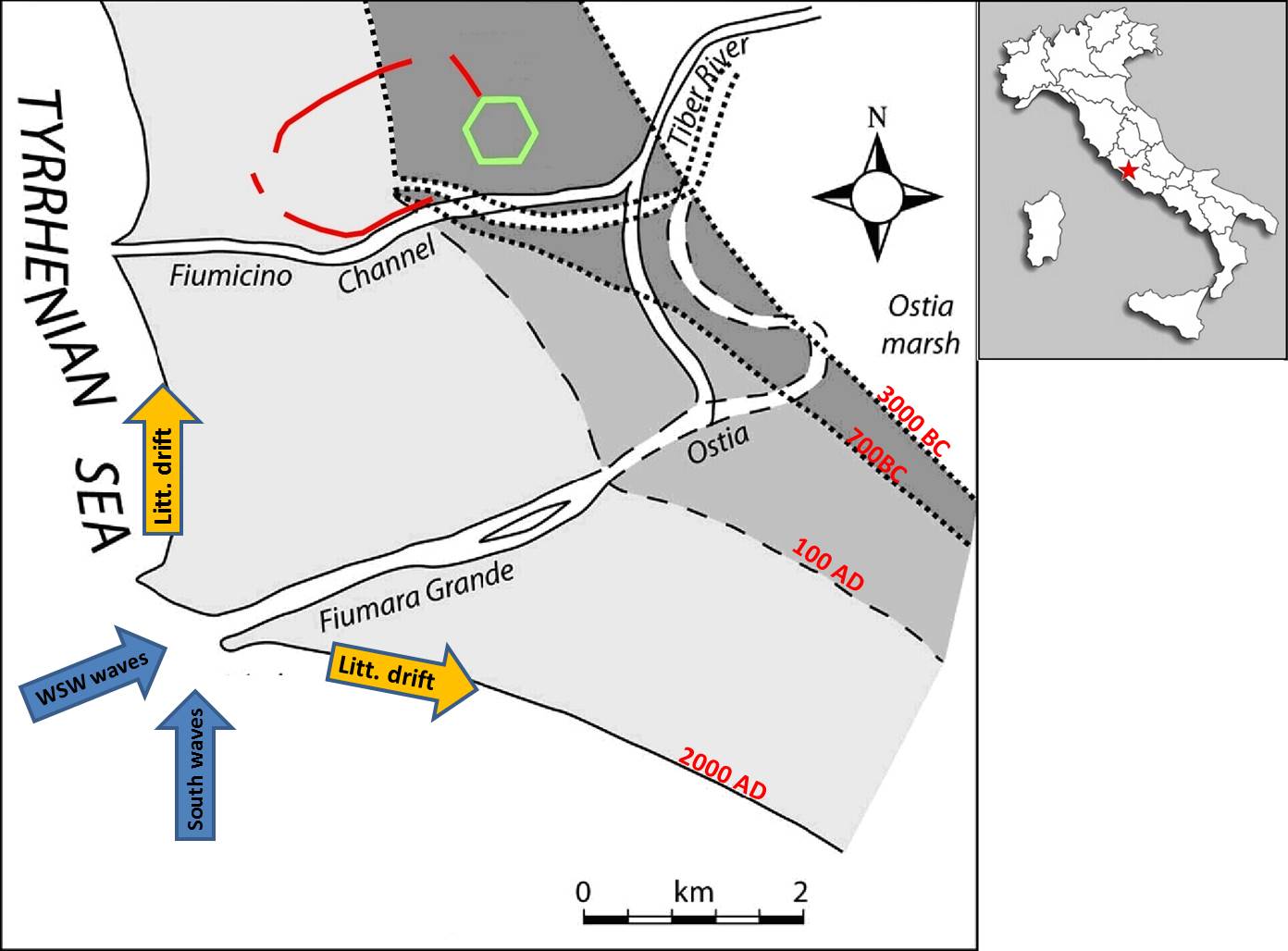
The picture above (based on P. Bellotti’s, 2011 study[2], see also Giraudi, 2009[12]) shows that the Tiber outlet moved from the north (into the future Roman ports) to the south (close to future Ostia), probably around the 7-8th century BC, before Ostia developed in the 5th century BC. It also shows that the shoreline between the present Fiumicino Canale and Fiumara Grande progressed 3.5 to 4 km between 100 AD and 2000 AD. That is an average close to 2 m/year. A more detailed analysis shows that this value might vary locally and reach 5 to 10 m/year near both outlets (Bellotti, 2011).
Further information is provided by Antonia Arnoldus-Huyzendveld and in her 2016 publications on coastline evolution[7a] and on Claudius harbour[7b].
Waves are dominant from SE to SW according to data taken from the Wind and Waves Atlas of the Mediterranean Sea (2004) at locations 42°N-11°E (west of Civitavecchia) and 41°N-12°E (south of Fiumicino).
Considering the local coastal morphology, the fluvial sediment load from the Tiber is supposed to flow as a littoral drift on both sides of the outlet, and offshore. The present total sediment load is 0.3 million ton/year (Milliman, 2014[3]) (that is around 150 000 cubic meter/year). It must be noted here that this fluvial sediment load was drastically reduced by a factor thirty (30!) during the 20th century due to upstream dam building. Anyway, the finer fraction (silt) flows offshore and only the coarse fraction (sand) remains in the coastal area (estimation of 50 000 to 100 000 cubic meter/year over the past centuries). The south breakwater of Portus Claudius obviously was a large obstacle to sediment movement towards north and sedimentation took place on the south side of the south breakwater.
Let’s see this in a simplified vertical cross-section placed just south of the south breakwater (looking north), and just after its completion.
NB: Roman Sea Water Level is around 0.8 m below present SWL[4]
(sketch distorted and not to scale)
Sediment from the prograding beach will start to get around the toe of the breakwater (BW) after a distance of 700 m. Sedimentation will start inside Portus Claudius at this moment. In the simplified scheme shown above (1:30 slope on a 10 m water depth, note that Morelli found 15 m[5]) and considering the 5 to 10 m/year progradation, the beginning of harbour sedimentation would be expected after 70 to 140 years, say one century, and that is well after Trajan decided to build his Portus Trajanus. This leaves many more years for the harbour to be still (partly) operational, as long as the water depth is at least 4 to 5 m inside the harbour. This seems to have been the case until at least 879 AD (Paroli[6]). We would consider nowadays that this is fairly overdesigned …
It would however not be surprising that Claudius’ engineers anticipated this, at least in a qualitative way, and this would then explain why they built such an expensive, long and deep, south BW, as they did not need a 10 m water depth for contemporary ancient ships, but they had to create a large sedimentation trap outside the harbour.
In the same line of thought, Claudius’ engineers may also have decided to use the concept of an arched breakwater on the northern side of the port, as this concept was already in use at Puteoli, Nisida and Misenum (by Agrippa in the thirties BC) for around one century. Such an arched breakwater was supposed to allow currents to flow through the breakwater, providing some flushing which would possibly help reducing siltation (modern engineers do not agree any more with this idea, see section on ‘silting up of harbours’).
Hypothetical sequence of breakwater construction
According to Dio Cassius (Roman History, 60, 11, transl. in Oleson, 2014, p 33) “First, he [Claudius] excavated a considerable plot of land near the coast, built quay walls all around it, and let in the sea. Next, in the sea itself he laid down great moles on either side of the basin entrance and thus enclosed a large body of water, and in it he fashioned an island carrying a lighthouse”. Hence, Claudius clearly built Portus in two stages: first inland near Monte Giulio as modern archaeology has recently shown (Arnoldus-Huyzendveld[7]), and second, both large breakwaters built into the sea.
If Claudius’ engineers realised that sediment coming from the Tiber was flowing north along the coastline as littoral drift, they must have thought that they had to build the south BW first in order to stop this material from settling inside the future harbour area against the northern BW, if that one were built first. They may not have realised that if sedimentation was to occur on the south side of the south BW, then erosion was to occur on its north side, i.e. inside the future harbour … That was quite a nice opportunity to let Nature do the work of cleaning up the area that would have to be dredged anyway … After some time, they would decide to start building the north BW and the coastline would readjust with some erosion near the northern side of the south BW combined with some sedimentation near the southern side of the north BW. The coastline between both breakwaters would then be stabilised. No problem so far.
However, as sedimentation on the southern side of the south BW continued, erosion had now to occur on the northern side of the north BW and this would soon start to undermine the landward end of the brand new north BW.
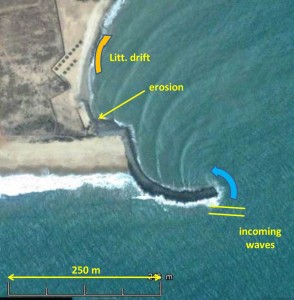
This picture shows the erosion area east of Cotonou where diffracted waves turn around the breakwater head, then follow the curved breakwater and take sand away at the landward end of it.
Portus’ configuration is reversed: waves follow the breakwater on the north side and “try to enter” the port from north to south by getting around the landward end of it, while sand is taken away further north.
This may be an explanation for the somewhat hectic layout of the north BW near Monte Arena[8], where several designs are used, possibly showing repair actions. A northern access channel for ships[9] may not have been anticipated from the onset by Claudius’ engineers, but the opportunity provided by this local erosion may have been taken to use it, and even to enhance it artificially, for river transit from Portus Claudius through the northern canal leading to the Tiber.
In the meantime, fine marine sediment was driven into the sheltered harbour area not only by residual waves behind the breakwaters, but also by small sea level variations such as those due to barometric variations, tidal effects and wind action. This fine sediment is therefore now found underneath coarser fluvial sediment that entered the harbour much later, coming from Fiumara Grande and drifting north along the coast to the harbour entrance.
These processes are summarised on the following hypothetical geomorphological evolution of the Portus Claudius area:
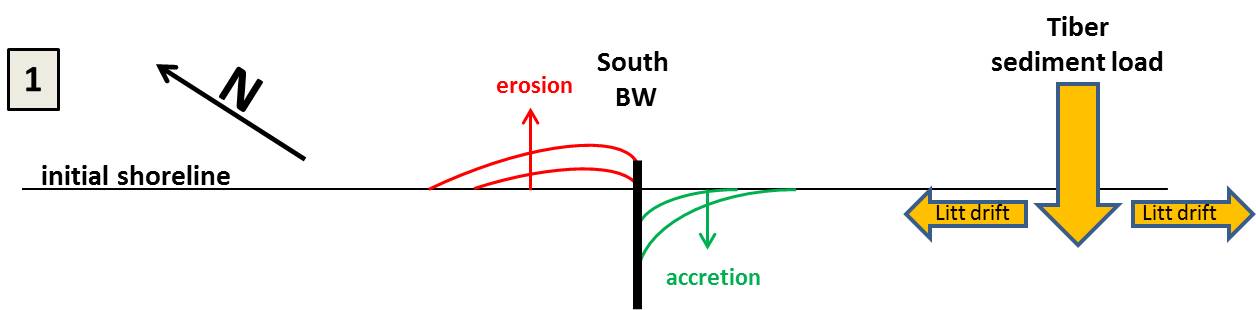
1) Construction of first breakwater (south)
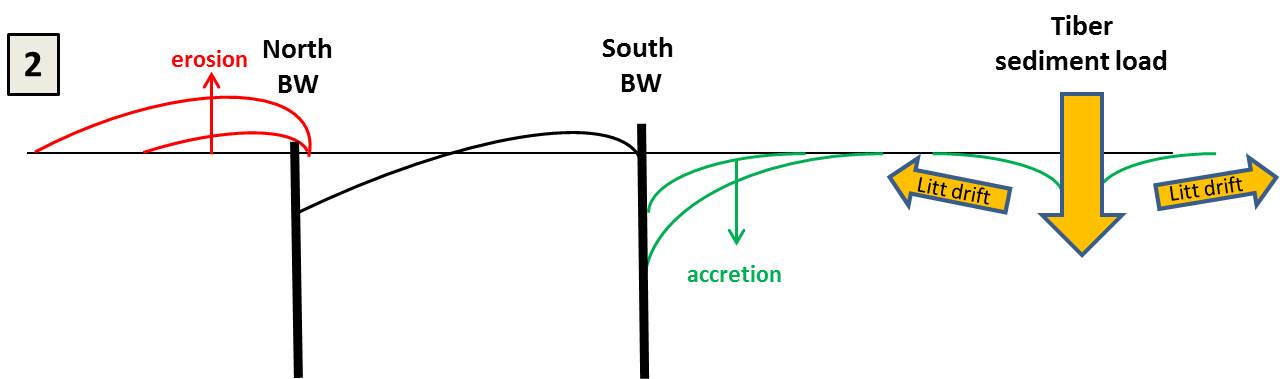
2) Construction of second breakwater (north)
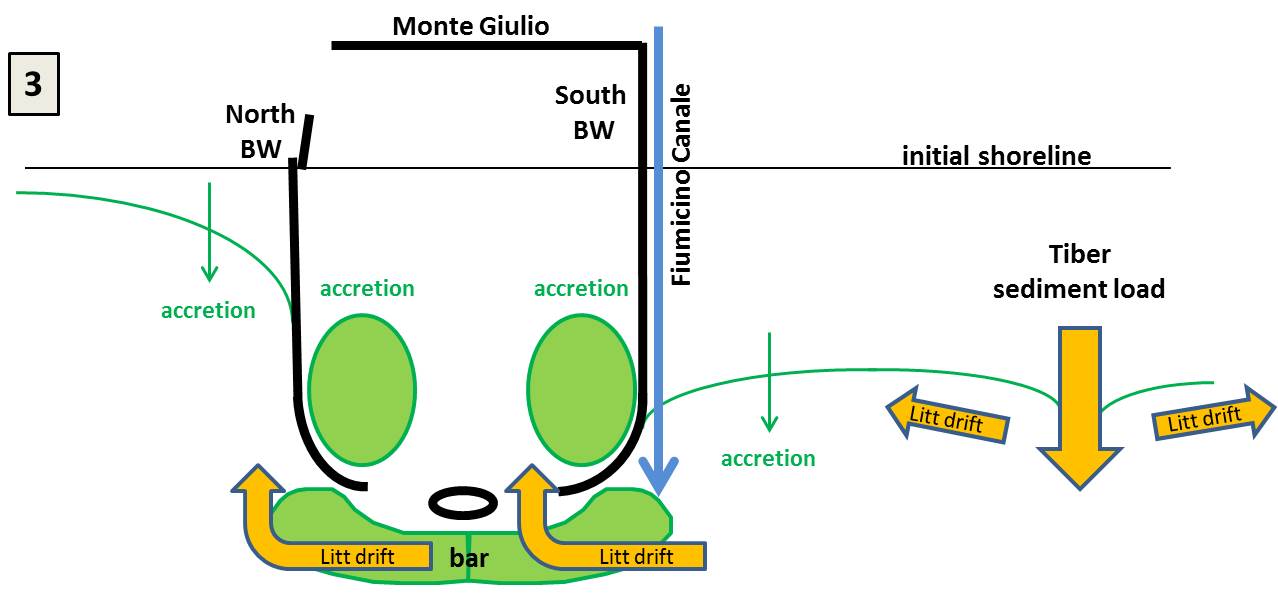
3) Coastal progradation and harbour sedimentation
Note that the so-called “Iseum” located just south of the via della Scafa viaduct over the Fossa Traiana (Lat 41.7727°, Long 12.2554°), was most probably built later than the Portus Claudius south breakwater. Hence, sedimentation on the south side of this breakwater was already progessing and the temple could be built on the new beach.
Fiumicino Canale – Fossa Traiana
Let’s stay on this southern side, where it remains to be explained how Fiumicino Canale could survive with such a large volume of sediment drifting to the north from the Fiumara Grande outlet. Many centuries after the Tiber outlet moved from the north to the south, Fiumicino Canale was artificially dug in the 1st century AD and later wrongly called “Fossa Traiana”. It provided a short connexion between the port (via Canale Trasverso) and the upstream river portion leading to Rome. Although this canal is the shortest way for the Tiber to sea, it was narrower than the branch flowing to Ostia and therefore did not attract a lot of river discharge water (and sediment). It is said that nowadays, the discharge ratio is 20% via Fiumicino Canale and 80% via Fiumara Grande, but that may have been very different at times (droughts, floods). A small hydraulic power of Fossa Traiana would not enable to keep its outlet open against massive sedimentation coming from the south and it seems likely that the outlet was closed periodically (if not permanently) near the landward end of the south breakwater, downstream of the Portico Claudio.
Rutilius’ observation[11] shows that such variations could happen, as in his time it was safer to sail out to sea via Fossa Traiana than via Ostia where a dangerous ‘bar’ had probably formed. He also states that they spent the night inside the port. As he does not mention a direct connexion of Fossa Traiana with the sea via a separate outlet, he might have sailed out to sea directly from Portus Claudius.
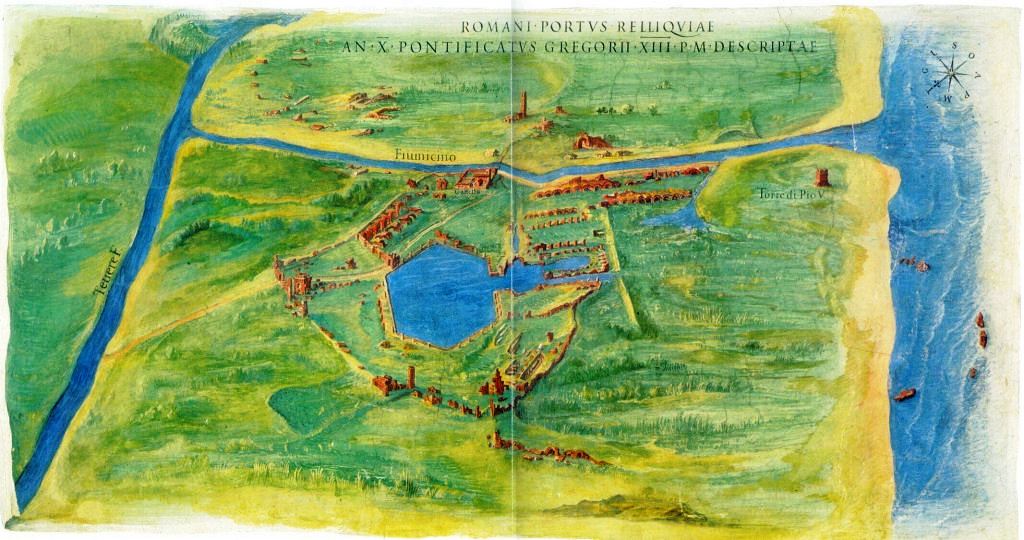
Paroli[6] tells us that Fiumicino Canale remained navigable certainly until 1118 AD, but that it was closed in 1461. However, Danti’s famous fresco shows an open Fiumicino Canale in 1582! His picture is quite accurate, showing various port remains, including in the sea, and we have no reason to doubt that the Fiumicino Canale was correctly drawn.
To achieve this, a training wall (e.g., rubble mound running parallel to the south breakwater) would be required to keep the outlet free from sedimentation and such a structure was not found by archaeologists, but it was perhaps destroyed by port development in 1612 inside Fiumicino Canale when it was re-opened towards the sea (Paroli, 2005[6]).
On the other hand, the Tiber being known for its strong floods (up to say 2000-3000 m3/s), it might be accepted that Fossa Traiana was periodically swept by such floods which would clean up the canal and enforce an opening to the sea at least once a year (possibly with some human assistance). By the way, a low sill (e.g., 1 m high) would help to prevent bed load sediment from penetrating into Fossa Traiana. The modern-day shape of the intake of Fossa Traiana on the Tiber at Capo Due Rami seems to confirm that special care is taken there:
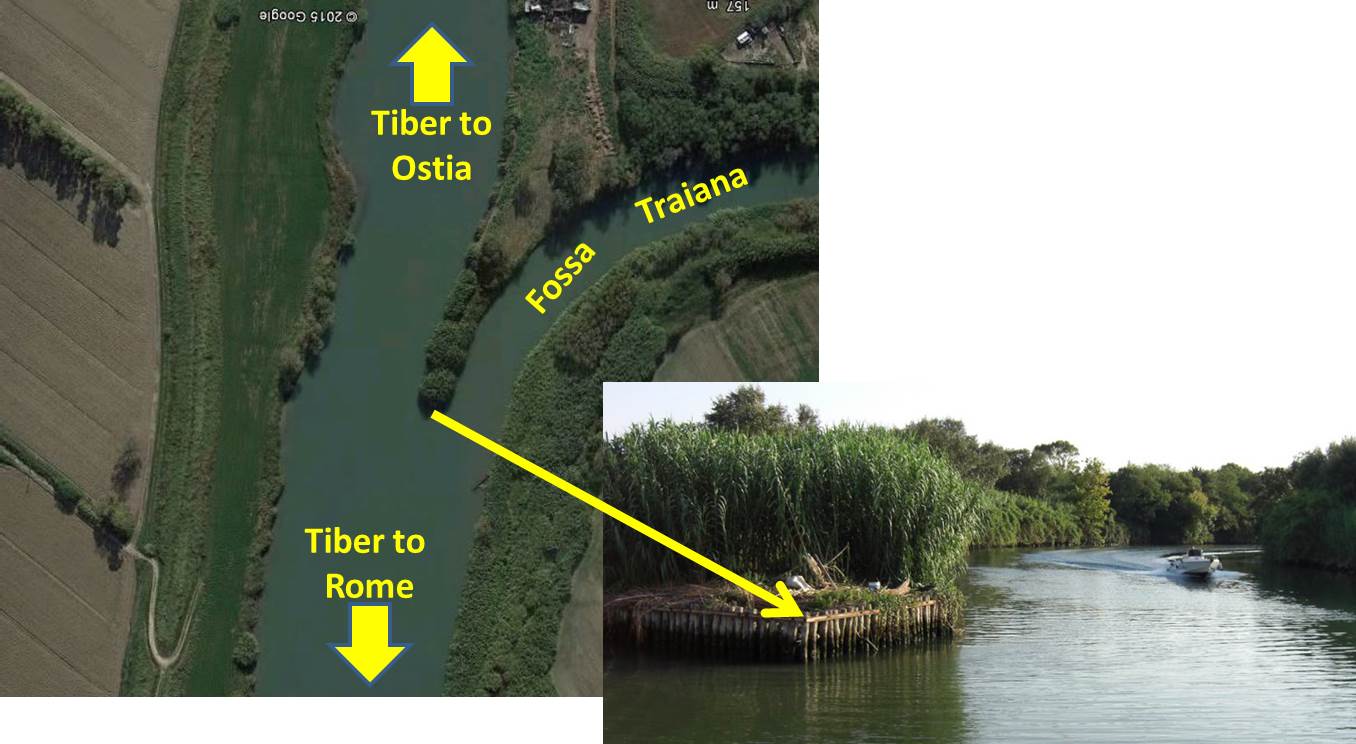
The intake structure is obviously calibrated to divert a certain fraction of the flow. It is reinforced in order not to be moved around by erosion. This arrangement may have been inherited from an ancestral (Roman?) tradition.
We are thus left with uncertainty as to the opening of the sea outlet
of Fiumicino Canale between 1118 AD and 1612 AD …
.
Summarizing the morphodynamics in the Portus area: sand brought by the Tiber was spread along the coastlines north and south of its outlet. The south BW of Portus Claudius stopped the littoral drift to the north inducing: a) sedimentation south of the south BW, b) closure of the seaward outlet of Fossa Traiana, and c) erosion north of the northern BW. After around one century, sand started to enter Portus Claudius by its main access channel, probably settling near the entrance, while finer materials entered further inside the port. Later on, sand bypassed the port entrance and spread on the coastline north of the port. Even later, the port was filled with sand and the coastline prograded in front of it.
Claudius’ breakwater remains
Engineers usually distinguish vertical breakwaters (BW) and rubble mound BWs. The first are built with caissons filled with hydraulic concrete (e.g., Caesarea Maritima, Israel). The latter are built by dumping stones from a lorry, and concrete can possibly be found on top of the rubble mound (above sea level where it is easier to pour); as we still do today (see http://fr.wikipedia.org/wiki/Brise-lames):
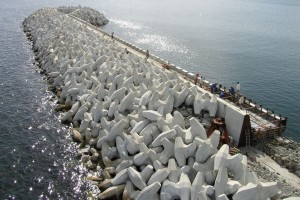
This picture (Fujairah) shows a modern BW under construction: large artificial blocks of concrete are used nowadays instead of rock, they are placed on top of, and as an armour layer of, a rubble mound made of quarry rock of several tons, which are themselves placed on a core made of quarry run. The crest structure (under construction) has a kind of “L” shape.
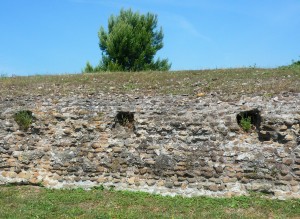
Morelli’s report on corings[14] show that the crest of the deep section parts of the breakwaters are located at approx. 5 m below present Sea Water Level (SWL) (i.e. 4 m below Roman SWL) with a total remaining structure height of around 10 m reaching approx. 15 m below present SWL. The initial BW may thus have been a 15 to 20 m high structure. We thus have two options: it could have been built higher and been partly destroyed by long term wave action, or have been built as a low crested BW from the onset. The first option is usually built as an emerging BW, built out from land with lorries, involving considerable logistics (lorries meeting each other on top of the BW, etc.). In the second option, building a BW that does not reach the water surface is done with barges from the water surface (like Pliny the Younger described at Centumcellae/Civitavecchia), and consequently the remaining upper level of the BW is built out from land with lorries, with ashlar blocks and/or possibly, with hydraulic concrete poured into wooden formworks to create a massive or arched structure. In any case, the upper level of the Portus breakwaters has been lost over the years: possibly due to re-use of stones during the Renaissance … or possibly due to wave action.
Let’s consider the latter case and assume (until further data is made available) that the deep section of the breakwaters consists of a rubble mound with an average stone diameter of 0.50 m.
We know from coastal engineers that because of wave breaking, waves cannot be larger than around 0.6 times the local water depth; hence in shallower water, waves are smaller and the required rock size for a stable BW is smaller too; conversely, a BW must thus have an increasing rock size when building out to sea on increasing depth. When we move into even deeper water, say over 10-15 m, breaking waves (of over 6-9 m) will not occur often, but just during storms; however, we may consider that any size of big storm will have occurred during the past 2000 years: so, if the water depth allows big waves to exist, they will occur in the long term and destroy the BW accordingly.
Clearly, 0.50 m rock (typically a 2 to 500 kg class of rock) is not stable with waves larger than only 1 m, which occur many times a year.
This is valid for frontal wave attack (wave crests parallel to the axis of the BW). Most of Portus’ BWs are not subject to frontal wave attack, but to (very) oblique wave attack, which is far less destructive. It is nevertheless expected that this 0.50 m rock placed on a water depth of 10 to 15 m should suffer frequent damage during storms, especially at the roundheads and at the lighthouse island which are both subjected to frontal wave attack.
Coastal engineers tell us that a rubble mound will be lowered by repeated wave attack until it is no more than a submerged breakwater. Its elevation above the seabed depends on the size of rock (see Failure of rubble mound breakwaters in the long term). In the case of Portus, with a water depth of 15 m and a rock size of 0.50 m diameter, the crest of the submerged BW would be lowered to 13 m below the water surface, i.e., 2 m above the seabed if waves were strong enough. But this is not the case in the area around Portus.
In addition, the total volume of rock cannot change. Hence, if a BW is flattened out by wave action, rocks must be spread over the seabed in the following way (with Roman water levels):
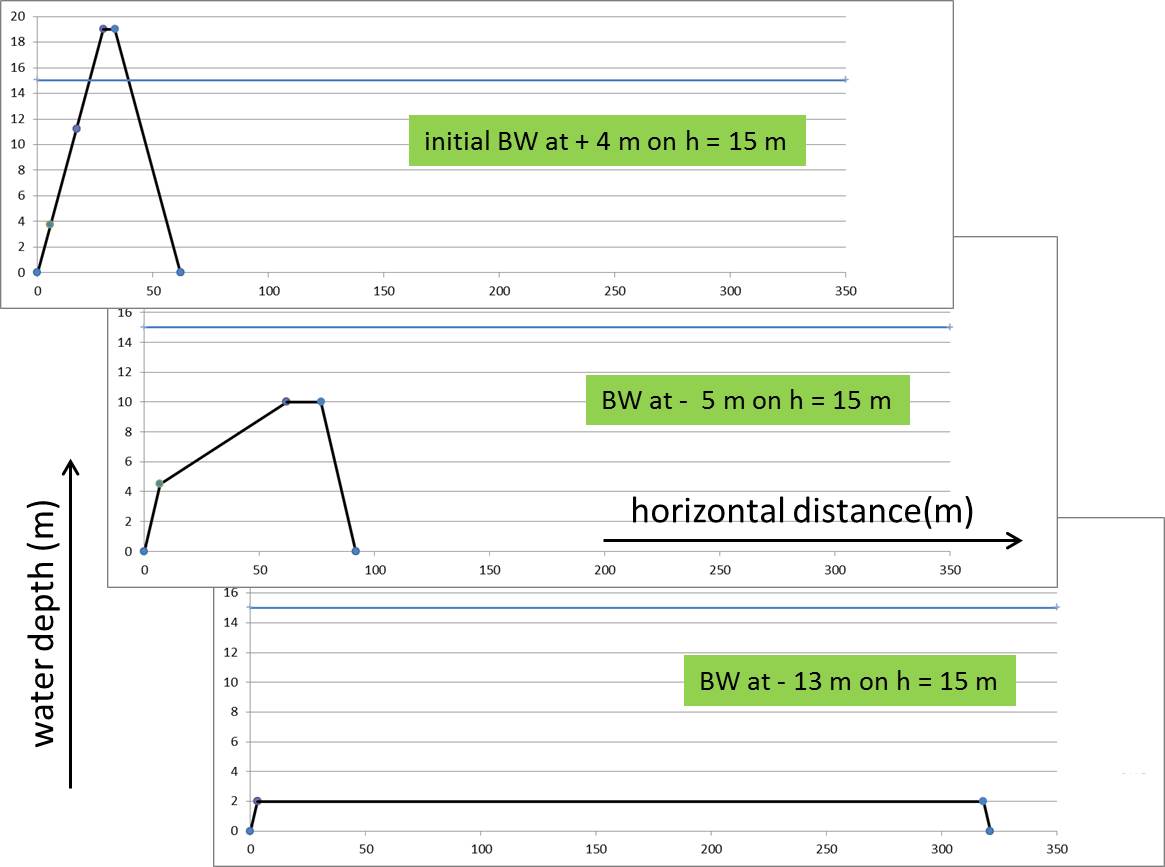
This is however not (yet?!) confirmed by archaeology … and large blocks of hydraulic concrete were not (yet?!) found either … It therefore seems more likely, at this moment, that the north BW was not made entirely of rubble, but that another structure (concrete or ashlar? massive or arched?) was built on top of a rubble mound having its crest at a few meters below Roman SWL. If this structure was not destroyed by wave action, ashlar blocks could have been dismounted during the Renaissance. The structure would thus have protected the underlying rubble mound from wave action for at least 1400 year until they were removed. After that, the rubble mound would be exposed to wave action and partly destroyed (see Failure of rubble mound breakwaters in the long term). This would explain why rubble was recently found on top of a thick harbour sedimentation layer[7b].
As a very temporary conclusion on the northern breakwater, four sections can be distinguished (see also Google Earth: https://www.ancientportsantiques.com/the-catalogue/italy/ ):
- Eastern end of the emerging breakwater, 425 m long, in the eastern part of which Oleson (2014)[19] made corings POR.2002.01 & 03, showing poor quality hydraulic concrete, possibly resulting from repair actions in this area further to local erosion and a temporary 200 m wide northern port access (Goiran, 2011[20]) ; further west, good quality hydraulic concrete was poured into wooden caissons from the seabed up to 2.5 m above the Roman SWL, and still visible on land ;
- Central part of the emerging breakwater, 333 m long, where travertine blocks were found up to around 2 m above the Roman SWL ;
- Western part of the emerging breakwater, 75 m long, ending near Goiran (2011) corings CL3/4, where hydraulic concrete and tuffo blocks were found by Testaguzza (1970) ;
- Submerged western section, about 900 m long, where Morelli (2011)[14] made corings PL04/05 and many others, showing rubble without any hydraulic concrete from the seabed at 13 m below Roman SWL, up to 4 m below Roman SWL, and a possibly disappeared upper layer, possibly arched and made of ashlar.
Testaguzza (1970) identified the three emerging parts of the ancient breakwater, but he did not find the submerged western section that was burried deeper than he could excavate at that time.
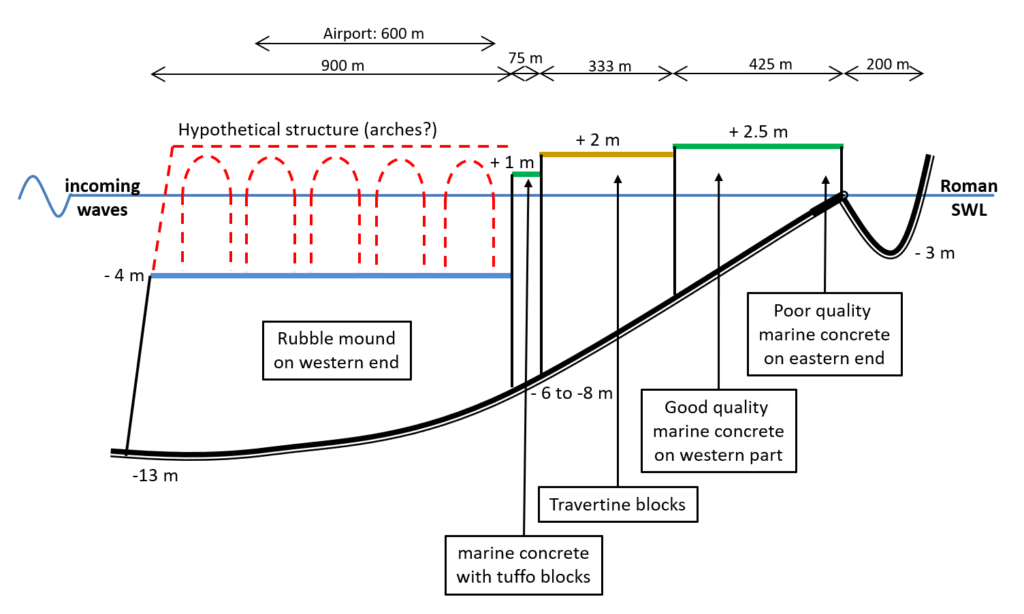
Next enigma: where are the arch-blocks??!
How safe was Portus Claudius?
Tacitus (Annals, 15, 18) reports that 200 ships were sunk inside the port during a storm in 62 AD. Some believe that this event was a tsunami, although no sedimentological evidence has been found so far to support this hypothesis[25]. In this study, we will show that a somewhat exceptional storm may also have induced this catastrophic event.
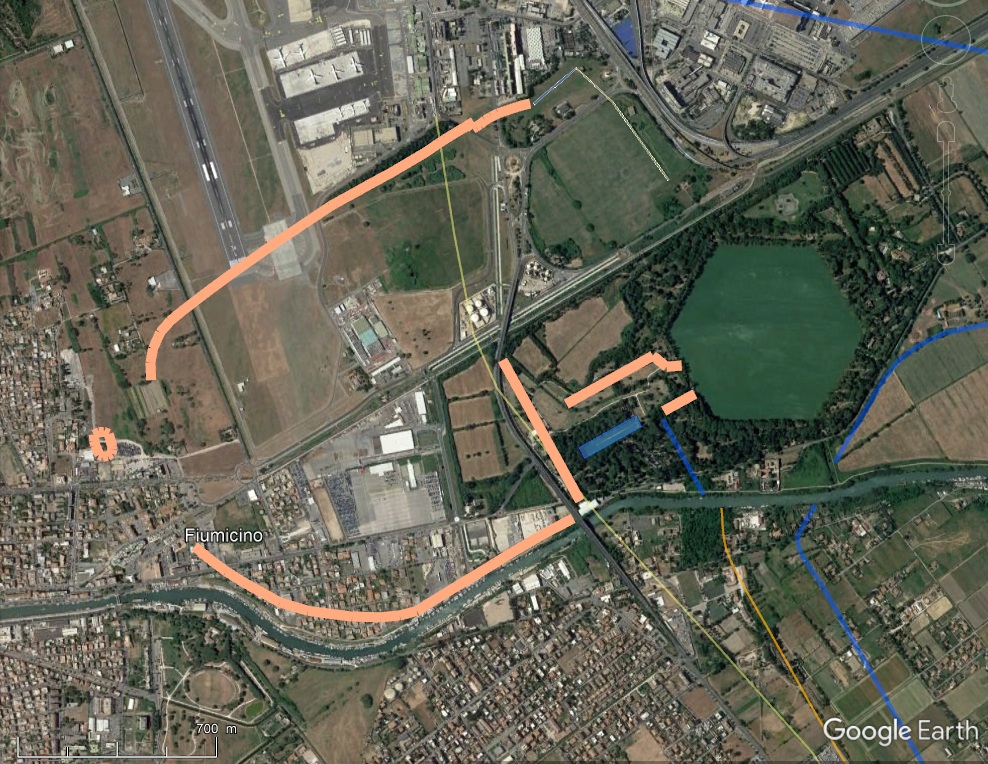
Note that only a small part of the island supposed to protect the harbour entrance has been located.
The remains of the main north and south breakwaters of Portus Claudius shown on the picture above leave a large harbour entrance for both ships … and waves. This wide opening is supposed to be sheltered by the offshore island which was only partly located by archaeology.
Obviously, the breakwater layout must be an optimum between limited wave penetration on one hand, and easy (wide) access for ships on the other hand. Ships may then shelter behind the main breakwaters, depending on the wave direction: ships may shelter behind the north breakwater with northern waves, and behind the south breakwater with southern waves. This is satisfactory with stable meteorological conditions. However, should the wave direction change from W to S and to E, or the other way round, dangerous situations for ships anchored inside Portus might occur because of wave directions turning around the harbour entrance. A sudden change could even generate a serious problem for ships anchored inside Portus, if it happened within a short time like one hour, because sailors would not have enough time to move their ships to a better sheltered area inside the harbour.
Wave penetration inside the port
Back in 2009, Noli and Franco performed wave penetration computations inside Portus Claudius, based on its assumed configuration[26]. Results from their work are shown here for waves from SW (220°), W (270°) and NW (310°). The protection provided by the (350 m) island compared to the (250 m) port opening determines the wave climate inside the port. As a result, very few waves penetrate inside this layout of the port, except for the NW sector of waves.
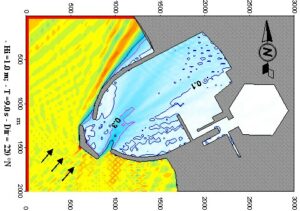
for wave direction of 220° (Noli & Franco, 2009).
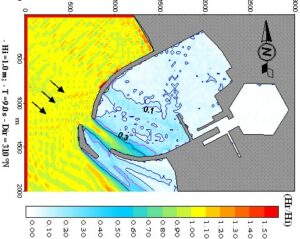
for wave direction of 310° (Noli & Franco, 2009).
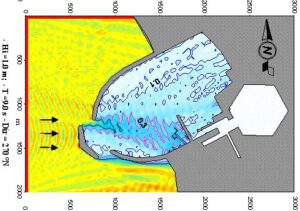
for wave direction of 270° (Noli & Franco, 2009).
A sheltered anchorage was thus provided behind the southern breakwater, say around 20 hectares, enough for say 200 ships at anchorage. However, should waves suddenly change their direction from the usual W – S sector to a NW sector, then the south anchorage area would be exposed to heavy wave penetration. In order to find out if such meteorological conditions could occur, we have to analyse the wave climate.
Waves in the Tyrrhenian Sea
As Murray (1987) has shown that the ancient wind climate is fairly close to the present one[27], we are going to use modern waves statistics for this study.
Wind waves are generated by wind blowing over the sea surface for a certain lapse of time and over a certain distance. During a storm, waves are thus generated under the wind field and propagate from there in the same direction as the wind. If the wind stops, the waves continue their trip with rather small loss of energy and some waves travel hundreds (even thousands) of kilometres outside their initial wind field. Such waves are called swell. This complex phenomenon is rather well understood today, enabling engineers and meteorologists to operate mathematical models predicting the wave climate in a certain area.
If we wish to understand waves, it is useful to understand how meteorological depressions travel over land and sea. In western Europe most depressions travel from west to east at variable speed. The winds that are associated with a depression usually flow in a counter-clockwise direction (in the northern hemisphere). In the Tyrrhenian Sea, depressions frequently stop and deepen in the Gulf of Genoa before moving on to SE. Hence, a depression travelling along the Italian mainland generates western winds (Libeccio) on its southern edge in the Tyrrhenian Sea. If such a depression travels more south, it generates southern winds first (Scirocco), followed by eastern winds, and possibly even northern winds, later on. This is of course a simplistic representation, aiming at clarifying this vast subject.
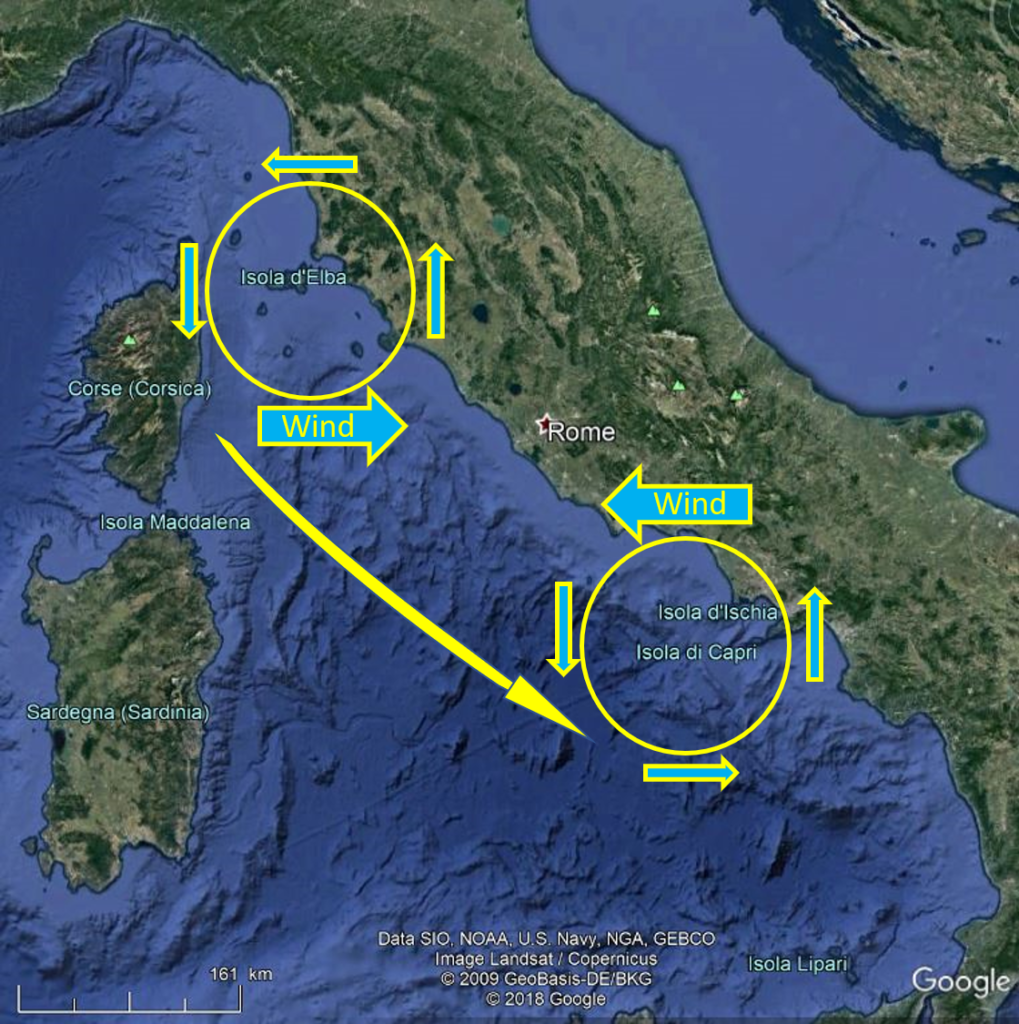
In the Tyrrhenian Sea, the waves can really approach the coast near Rome only from a sector from west to south because other wave directions such as SE and NW provide waves that propagate more or less parallel to the coast. Such waves will bend towards the coast sooner or later (due to refraction) while losing much of their energy. Wave statistics computed for Ostia confirm that most large waves come from a WSW direction, with some smaller waves from south.
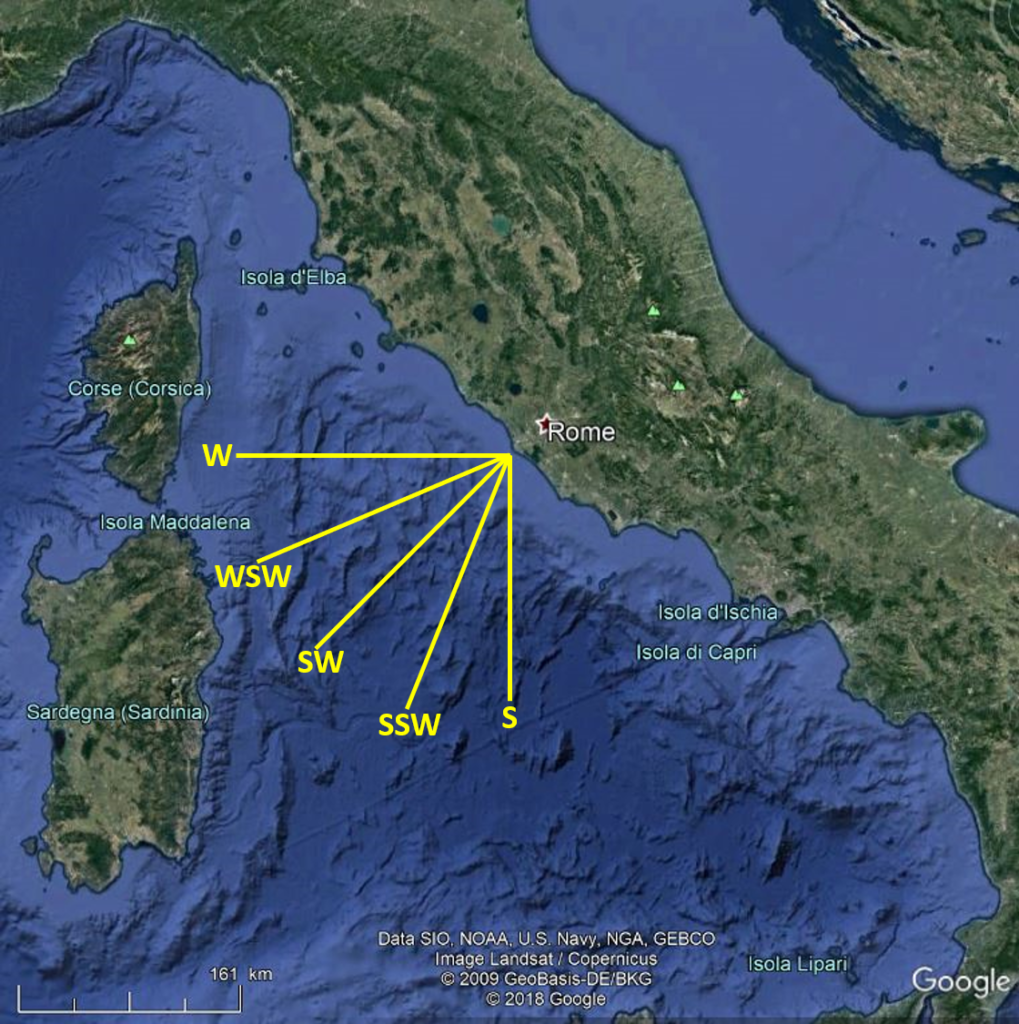
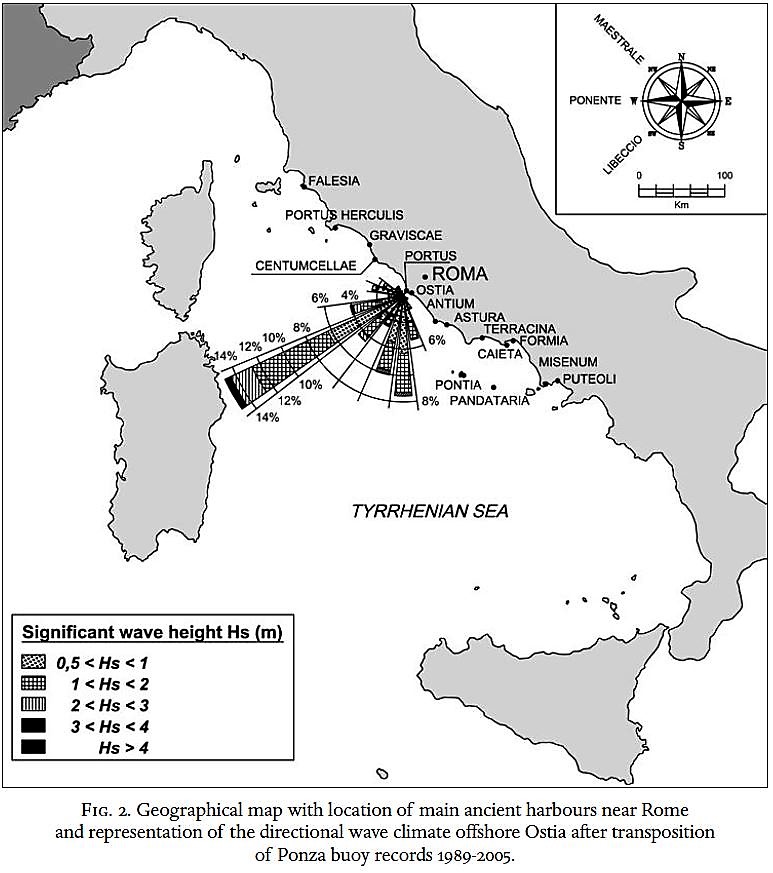
Analysis of wave data from a buoy near Ponza (ancient Pontia)
Our objective is to identify individual storms and identify sudden wave-direction reversals during each storm, if any.
The buoy is located at 40.866°N, 12.950°E, on 115 m water depth, offshore between Rome and Naples. Its registration period is 1989-2008, but the first period until end of 2002 provided records only every 3 hours which is not detailed enough for our purpose. In the period of 2003, the measurement of wave directions was not satisfactory. A new (Triaxys) buoy was apparently installed early January 2004 and a coherent set of 43876 records from 22/1/2004 to 30/3/2008 was chosen for the present analysis.
The data is recorded every 30 min providing Hs (significant wave height in m), Dir (wave direction in degrees to north), Tp (period of the peak of the wave spectrum in s), Tm (mean wave period in s). The sampling is at 1.28 Hz on each 20 min time-series, which means that a 6 s wave is described with 7 to 8 points, and that around 200 waves are analysed for each 20 min record.
A storm in this area can be defined as Hs > 1.5 m. It may be called a “large storm” when Hs = 4 m and more. The storm durations are taken as Hs > 1.5 to 2 m. A change in wave direction is called “sudden” when it occurs within 30 min.
Obviously, the waves from N and from E that are registered at Ponza, do not exist or are very small at Portus. Wave-direction changes from W to N, or from S to E are thus favourable for ships anchored inside Portus, as waves reduce during such an event, even if the wind may remain a problem.
The following results were found:
- Identified storms:
28/2/2004, 1.5 days, Hs = 4 m, Dir 225° => 270° (gradual change of 45° over 24h)
24/3/2004, 1.5 days, Hs = 4 m, Dir 270° (stable)
25/9/2004, 1.5 days, Hs = 3 m, Dir 270° => 360° (gradual 90° change)
8/11/2004, 1 day, Hs = 2 m, Dir 115° => 315° => 115° (two sudden 200° reversals)
20/11/2004, 1 day, Hs = 4 m, Dir 270° => 90° (sudden 180° reversal)
25/1/2005, 1 day, Hs = 2 m, Dir 270° => 90° (sudden 180° reversal)
4/8/2005, 0.5 day, Hs = 1.5 m, Dir 295° => 90° (fast 155° reversal)
8/8/2005, 0.5 day, Hs = 1.5 m, Dir 270° => 180° (fast 90° change)
27/9/2006, 0.5 day, Hs = 1.5 m, Dir 250° => 360° => 270° (two sudden 90° changes)
2/11/2006, 1 day, Hs = 3 m, Dir 270° => 45° (sudden 135° near-reversal)
2/1/2007, 1 day, Hs = 4 m, Dir = 270° (stable)
24/1/2007, 2 days, Hs = 4-5 m, Dir = 220° => 270° (gradual change of 50° over 36h)
28/5/2007, 1.5 days, Hs = 4 m, Dir = 270° (stable)
21/10/2007, 2 days, Hs = 2 m, Dir = 115° => 360° => 295° (one sudden 245° reversal, one sudden 65° change)
8/12/2007, 3 days, Hs = 4 m (twice), Dir = 270° (stable)
23/1/2008, 1 day, Hs = 1.5 m, Dir 270° => 70 ° (sudden 160° near-reversal)
- We found 7 large storms with max Hs = ca. 4 m during the registration period of ca. 4 years, and that is an average of 1 to 2 large storms per year, but 3 large storms were found in 2004 and in 2007, and none occurred in 2005 and 2006. All large storms (save one on 28/5/2007), occurred during the winter months (November – March).
- The storm durations are between 0.5 and 2 days (save one in 2007 lasting 3 days).
- For large storms, the mean wave period Tm = 7 to 9 s, which yields a wave length of 60 to 80 m on a 10 m water depth, and 45 to 60 m on a 5 m water depth. Smaller storms feature Tm = 5 to 6 s, which yields a wave length of 35 to 50 m on a 10 m water depth, and 30 to 40 m on a 5 m water depth.
- The dominant wave direction is from SW to W, both for small storms (Hs > 1 m) and for large storms (Hs > 3.8 m).
- Four large storms and many other storms show stable wave directions. Five storms show gradual wave direction changes.
Two gradual wave direction changes have been found (45° over 24 h on 28/2/2004 and 50° over 36 h on 24/1/2007) but it may be considered that this did not induce serious problems for ships moored inside the port.
One case with a somewhat faster gradual change in wave direction was found on 25/9/2004 when the change from W to N occurred within 2.5 h near the end of the storm when waves were decaying from Hs = 2 m to Hs = 1 m.
Even faster changes occurred within 1 h and 1.5 h on 4 and 8/8/2005 respectively. On 4/8/2005, the reversing from NW to E, via N, occurred in 60 min, with Hs = 1 to 1.5 m.
On 8/8/2005, the reversing from W to S occurred within 90 min when waves were Hs = 1.5 m.
- Sudden 90° to 180° changes in wave directions have been found 10 times during the 4-year period of observation:
On 8/11/2004, the change from E to NW occurred within 30 min at the beginning of the storm with waves were increasing from Hs = 1 m to Hs = 1.5 and 2 m.
On 8/11/2004, a sudden reversal from NW to E occurred at the end of the storm.
On 20/11/2004, the reversing from W to E occurred within 30 min near the end of the storm when waves were decaying from Hs = 3 m to Hs = 2 m.
On 25/1/2005, the reversing from W to E occurred within 30 min near the beginning of the storm when waves were Hs = 2 m.
On 27/9/2006, the change from WNW to N occurred within 30 min when waves were Hs = 1.5 m.
On 27/9/2006, 2.5 hours after the first change, waves turned further from N to W.
On 2/11/2006, the reversing from W to NE occurred within 30 min when waves were Hs = 2 m.
On 21/10/2007, the change from E to N occurred within 30 min when waves were Hs = 1.5 m.
On 21/10/2007, 3 hours after the first change, waves turned further from N to NW.
On 23/1/2008, the reversing from W to NNE occurred within 30 min when waves were Hs = 2 m.
- Out the 10 cases shown above, 4 show a sudden change from W to S and to E, corresponding to the usual path of meteorological depressions, as explained before. Several other cases show waves suddenly turning to N.
Only one case on 8/11/2004 shows a sudden change from E to S and to NW.
Imagine a storm like the one on 8/11/2004: the wave-direction change from ESE (115°) to NW (315°) occurs within around half an hour which is really short for sailors to move their ships to seek better shelter inside the port. Two or three hundred ancient ships are at anchor in the lee of the southern breakwater with a gentle eastern wind and almost no waves inside the port. Ships are using their own anchors or fixed mooring boxes placed on the seabed. The water depth is quite large (5 to 10 m) and the mooring lines are long. The ships’ sterns are located at 30-50 m from their anchor. Everything is under control and only one or two sailors remain on board of each ship for safety.
Now, suddenly, within half an hour, the wind and waves turn to NW, first with Hs = 1 m waves outside, then growing to 2 m within a few hours. The sheltered area in the lee of the southern breakwater now receives 0.5 m waves (growing to 1 m). The ships simultaneously turn around their anchors to align in the wind direction. No big problem so far. However, waves start to shake the ships who are pulling on their anchor lines and anchors will start to rip on the seabed. Anchor lines that are tied up to fixed mooring boxes may break.
That is the beginning of a drama … Unmoored ships are quickly blown towards the southern breakwater where they crash on each other. Such an event might end up in a drama like in year 62 when 200 ships were sunk in Portus Claudius.
Berthing capacity of Portus Trajanus
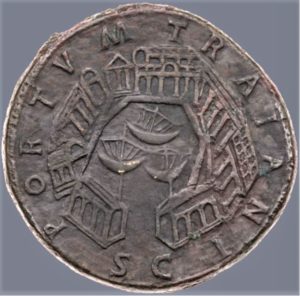
(source: www.ostia-antica.org).
Like today: Bread and games to ensure social peace …
(« Panem et circenses » Juvenal, Satires, 10.81)
Let’s try to think like a Roman port engineer in 105 AD …
Concerning the games, we have the Colosseum (built between 72 and 80 AD) and concerning bread, we need a harbour basin enabling us to ensure Rome’s supply of food. We already have Portus Claudius (around 200 hectares, built between 42 and 54 AD) but 200 ships were sunk in this port during a storm in 62 AD. Indeed, when observing the areas sheltered from waves in L. Franco’s computations[15], a sheltered area of around 20 hectares is found close the south breakwater for SW waves, and around 40 hectares is found close the north breakwater for western waves (NB: dominant waves are from SW to W). As around 10 ships of 25 x 7 m can anchor on one hectare of water area, it can be seen that around 200 ships could be anchored in Portus Claudius. That is quite a lot of ships but a disaster like the one in 62 AD could occur if the wind would suddenly change direction.
We therefore need to add a new basin with better protection from storms: the construction of Portus Trajanus (33 hectare) will be undertaken from 106 to 113 AD.
This new basin will combine very well with the existing Portus Claudius which has a large basin that can be used as an outer harbour allowing sailing in under full sail and furling sails in a sheltered area. This existing basin offers a shelter for around 200 ships at anchor while waiting for unloading. The new basin will not only offer better shelter against storms, but also have many warehouses and a new canal to the Tiber from where goods will be moved faster upstream over around 35 km to Rome on hauled barges. Traffic will be separated: sea-going ships on one side of the new basin and river barges on the other side near the new canal, with warehouses in between. This separation is still in use in some ports nowadays (e.g., Rotterdam) as it separates the marine world from the river world.
The logistic chain is thus completely redesigned.
Around 200 000 to 400 000 tons/year of grain coming from North Africa (Egypt, Tunisia) must be provided to feed the one million people of the city. Other goods must be added to this (olive oil, wine, garum, etc.). The total traffic can be estimated at 500 000 tons/year, as an average[16].
With 200- to 500-ton ships making two trips a year, 1000 ships are required to provide 2000 shiploads averaging 250 tons per load[23]. This is obviously quite approximate and variations around these figures can be thought of, e.g., a part of these shiploads might go through Puteoli[29] and further to Ostia by means of smaller coasters that would pass the sandy bar at the entrance of the Tiber, and probably even be towed all the way to downtown Rome.
Hence, let’s stick to 1800 shiploads/year transiting through Portus Trajanus.
These ships sail mainly (and not ‘only’) during the good season (early April to the end of October) using the “summer winds” from NW that blow on the eastern Mediterranean in July-August and allowing a fast trip from Rome to Alexandria (one or two weeks, but at least double on the trip back to Rome). A concentration of ships arriving at Portus may thus be expected before and after July-August, in June and in October.
As each 250-ton ship carries around 8 000 sacks of one artaba (ca. 39 l) weighting around 30 kg each, and if unloading was organised as a continuous file of individuals, it might be possible to unload a ship within a few days, but it is more realistic to expect around 10 days for unloading a 250-ton ship and to take in provisions and settle formalities[24]. If we wish to host 900 ships in June (first trip) and 900 ships in October (second trip), then we need a basin with quays for around 300 ships (i.e., 3 groups of 300 ships staying for 10 days each).
On the layout map of Portus Claudius[17] , 1000 m of quay walls are found outside the “Darsena” (the Darsena itself, 48 x 230 m, is supposed to be used by river boats). In addition, a further 700 m can be found along the Portico di Claudio (300 m) and Molo di Claudio (“Nord-Sud”) (2 x 200 m). The total quay-wall length available for large sea-going ships thus does not exceed ca. 2000 m. Hence, the total number of sea-going ships docked in Portus Claudius is limited to a maximum of ca. 100 ships, plus 100 ships on Portico di Claudio + Molo Nord-Sud, available only in good weather conditions.
Enlarging the port is therefore a necessity.
Harbour Basin Shapes
Let’s suppose we get a phone call from the emperor ordering the digging of a new harbour basin for 300 ships of 25 x 7 m … We would first need to provide a quay length of 300 x 7 m = 2100 m (all ships being docked bow first like on the Torlonia relief). Any basin shape might be accepted, from a straight-line of 2100 m to a circle with 668 m diameter, including a triangle, a rectangle, a hexagon, etc.
For all angular shapes, some length is lost in the angles if ships are not to hinder each other.
The circular shape would be tempting to reduce the volume of excavation, but the circular shape does not provide linear quays that are preferred for port operations.
Angular shapes have better perimeter/surface ratios. Let’s start with an isosceles triangle which offers 30% more perimeter for the same surface as a circle, but quite some length is lost in its sharp angles. Then come the square, the rectangle and multi-faced shapes like pentagon, hexagon, etc. and finally, the circle. The total length lost in the angles obviously increases with the number of angles, but at the same time the length lost at each angle reduces, and it is seen on parameter C below that both effects more or less compensate each other.
Let’s have a closer look at Portus Trajanus. It consists of a hexagon with six 358 m sides which is thus inscribed in a circle with a 716 m diameter. This hexagon has a perimeter of 2148 m and an area of 33.3 hectares. This seems quite close to what we need to berth 300 ships with a length of 25 m and a width of 7 m as it has a little more than the 2100 m of quay length we are looking for.
Let’s now go back to polygons with a 2148 m perimeter. We computed the number of ships that might be aligned bow or stern first side by side in polygonal basins with an increasing number of sides. We also computed the basin area and the number of ships per unit of area to be excavated.
N = total number of ships in the basinn = number of sides of the basin
a = length of each side of the polygon
L = length of ships (25 m)
b = width of ships (7 m)
D = diameter of the circle in which the polygon is inscribed
C = total quay length lost in the angles
P = perimeter of the polygon = quay length to be built
S = surface of the polygon = surface of the basin to be excavated
N/10 S = number of ships per 10 excavated hectares
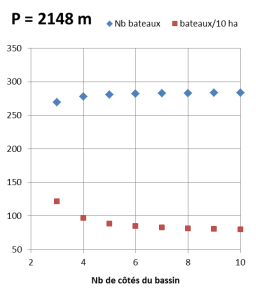
The number of sides of the polygon is set out horizontally and the number of ships in the basin is set out vertically. It can be seen that the number of ships does not vary much (around 280) with the number of sides. The triangle provides a little less quay length than the other shapes.
It can be seen that between 8 and 10 ships per excavated hectare can be hosted (except for the triangle which can host over 12 ships/hectare).
It must be noted that a linear basin consisting of only 2 long quays of 1000 m each would also host around 285 ships. The surface would be only around 10 hectares (assuming a basin width of 4 ship lengths), leading to 28 ships/hectare and to a much smaller volume of excavation.
As a conclusion, it can be said that for 2148 m of quays to be built (including a little less than 2000 m really available for docking), around 280 ships can be docked bow first in a basin with four or more sides. Obviously, a smaller number of larger ships would be docked in the same basin, e.g., less than 220 ships of 35 x 9 m, instead of 280 ships of 25 x 7 m.
A linear or a triangular shape would be optimal if the volume to excavate was to be minimised, but this approach was clearly not chosen. The volume to excavate was therefore not the main design parameter and it may be accepted that (like today) excavation in a sandy subsoil was relatively cheap compared to the cost of quay wall building.
The hexagonal shape is not particularly optimal from a point of view of number of berths or volume of excavation. It must therefore have attracted the Roman designers for other reasons:
- integration into the existing geography and land use,
- inspired by the famous circular ‘cothon’ at Carthage?
- with each of the six sides specialising on particular goods and warehouse types.
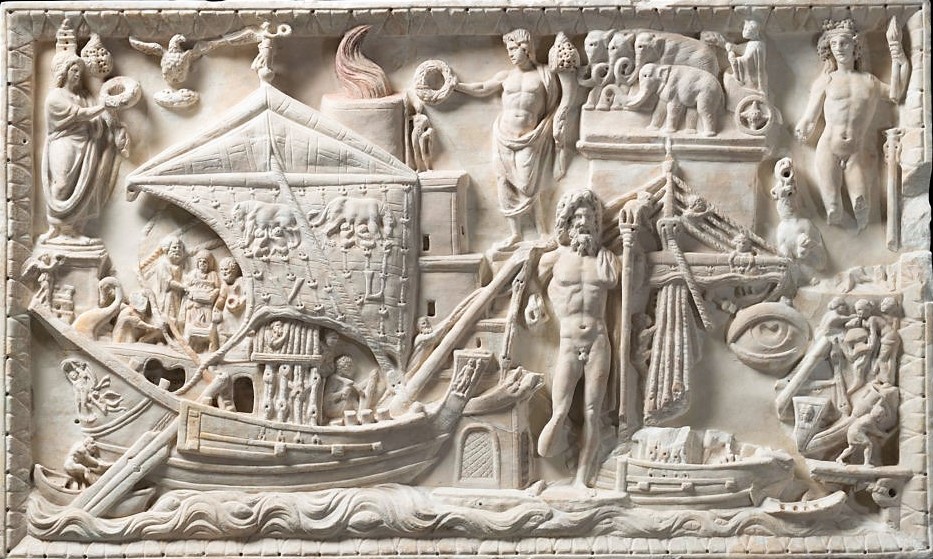
(photo credit: Zètema – Roma Capitale).
References
[1] BELLOTTI, P., 2000, “Il modello morfo-sedimentario dei maggiori delta tirrenici italiani”, Boll. Soc. Geol. It., 119 (2000), p 777-792.
[2] BELLOTTI, P., 2010, “The Tiber river delta plain (central Italy): Coastal evolution and implications for the ancient Ostia Roman settlement”, The Holocene, 21(7) p 1105-1116, Sage Publications Ltd.
[3] MILLIMAN, J., 2011, “River Discharge to the Coastal Ocean: A Global Synthesis”, Cambridge University Press, UK (384 pp).
[4] GOIRAN J.-P., et al, 2009, “Découverte d’un niveau marin biologique sur les quais de Portus: le port antique de Rome”, Méditerranée, 112, pp 59-67.
[5] MORELLI, C., 2011, “Porto di Claudio: Nuove scoperte”, in “Portus and its hinterland: Recent archaeological Research”, ed. Simon Keay & Lidia Paroli, The Britisch School at Rome, p 47-65.
[6] PAROLI, L., 2005 “History of past research at Portus”, in KEAY, S., & MILLETT, M., 2005, “Portus in Context”, The British School at Rome.
[7] ARNOLDUS-HUYZENDVELD, A., et al, 2015, “Il paleoambiente di Monte Giulio e della parte nord-orientale del bacino portuale di Claudio”, The Journal of Fasti Online, Associazione Internationale di Archeologia Classica (www.fastionline.org/docs/FOLDER-it-2015-324.pdf)
[7a] ARNOLDUS-HUYZENDVELD, A., 2016, “How the coastline of Ostia changed over the centuries“, Foro N° 41, Friends of Ostia, (13 p).
[7b] ARNOLDUS-HUYZENDVELD, A., 2016, “The hidden harbour“, Foro N° 63, Friends of Ostia, (9 p).
[8] FELICI, E., 2013, “Il Porto di Claudio e Vitruvio”, Atlante tematico di topografia antica: ATTA : rivista di studi di topografia antica, 23(2013), Roma: “L’ERMA” di BRETSCHNEIDER.
[9] GOIRAN J.-P., et al, 2008 “Portus, la question de la localisation des ouvertures du port de Claude : approche géomorphologique”, Mélanges de l’Ecole Française de Rome, 121, pp 217-228.
[11] RUTILIUS NAMATIANUS, 5th c. AD, “De Reditu Suo”, Book 1, Verse 179: “Then at length, I proceed to the ships, where with twy-horned brow the branching Tiber cleaves his way to the right. The channel on the left is avoided for its unapproachable sands […]. We hesitate to make trial of the sea; we tarry in the haven […]. In the half-dawn we weigh anchor, […], we make way along the nearest shores […].”
[12] GIRAUDI, C., 2009, “Late Holocene Evolution of Tiber River Delta and Geoarchaeology of Claudius and Trajan Harbor, Rome”, Geoarchaeology, Vol 24, N° 3.
[13] BARTOCCINI, R. 1958, “Il Porto Romano di Leptis Magna”, Boll. Centro Studi per la Storia dell’Architettura, N°13.
[14] MORELLI, C., 2011, “Porto di Claudio: Nuove scoperte”, in “Portus and its hinterland: Recent archaeological Research”, ed. Simon Keay & Lidia Paroli, The Britisch School at Rome, p 47-65.
[15] NOLI, A. & FRANCO, L., 2009, “The ancient ports of Rome: new insights from engineers”, Archaeologia Maritima Mediterranea, 6, 2009.
[16] REDDE, M., 2005, “Voyages sur la Méditerranée romaine”, Actes Sud, (p 44).
BRANDT, J., 2005, “The Warehouse of the World”, A Comment on Rome’s Supply Chain during the Empire, Orizzonti. Rassegna di archeologia 6 (2005), (p 25–47).
TCHERNIA, A., 2011, “Les Romains et le commerce”, Centre Jean Bérard, (p 275-287).
[17] KEAY, S. & MILLETT, M., 2005, “Portus in Context”, Portus, an archaeological survey of the port of imperial Rome, The British School at Rome.
BUKOWIECKI, E. & MIMMO, M., 2021, “Infrastructures portuaires à Portus – Les entrepôts dits de Trajan et le môle nord-sud”, Colloque Fréjus, 2018.
[18] KOMAR, P., 1998, “Beach processes and sedimentation”, 2nd ed., Prentice Hall.
[19] OLESON, J., BRANDON, C., HOHLFELDER, R., JACKSON, M., 2014, “Building for Eternity – The history and technology of Roman concrete, engineering in the sea”, Oxbow Books.
[20] GOIRAN, J-P., et al. 2011, “Caractéristiques sédimentaires du basin portuaire de Claude: Nouvelles données pour la localisation des ouvertures”, in “Portus and its hinterland: Recent archaeological Research”, ed. Simon Keay & Lidia Paroli, The Britisch School at Rome, p 31-45.
[21] MANGOR, K., et al. 2010, “Bypass harbours at littoral transport coasts”, PIANC MMX Congress, Liverpool.
[22] CUYLER, MJ., 2014, “Portus Augusti: The Claudian Harbour on Sestertii of Nero”, in “Art in the Round”: New Approaches to Ancient Coin Iconography, Tübinger Archäologische Forschungen, Band 16.
[23] ARNAUD, P., 2016, “Conclusion”, in “The Sea in History: The Ancient World – La Mer dans l’Histoire L’Antiquité”, Edited by Pascal Arnaud and Philip de Souza, General editor Christian Buchet, Woodbridge, The Boydell Press, (p. 623). See also section on ancient merchant ships.
[24] BOETTO, G., BUKOWIECKI, E., MONTEIX, N. et ROUSSE, C., 2016, “Les Grandi Horrea d’Ostie”, in “Entrepôts et trafics annonaires en Méditerranée”, Marin B. et Virlouvet C. (dir.), Ecole Française de Rome, 522, (p 177-226). This paper informs us that 3 days are needed to unload 70 tons, and refers to POMEY (1978) who speaks of 2 to 4 days to unload a small- to medium-size ship. Pomey refers to ROUGE (1952) who speaks of 2 days to unload and 4 days to load a ship of untold size. Rougé refers to WILCKEN (1912) who translates a letter of Eirenaios to his brother in Egypt, telling him that he arrived in Portus on June 30 and that his (probably large) ship was unloaded on July 12 (2nd or 3rd c. AD). Rougé also refers to ASHBURNER (1909) who translates a contract telling us that the captain has 4 days to unload 250 artaba (a small ship) in 236 AD.
In addition, KEAY, S., 2014, mentions 2 to 6 days for unloading a 150- to 350-ton ship (p 161). This order of magnitude was also confirmed by BRANDT (2005).
Note also that, depending on (possibly corrupt) friends the shipper has in the port, administrative formalities may take more time and require the ship to be anchored away from the quay walls.
[25] DELILE, H. & SALOMON, F., 2020, “Palaeotsunami deposits at the Tiber River mouth (Ostia Antica, Italy): Do they really exist?”, Earth-Science Reviews, EARTH 103268, Elsevier, (59 p).
[26] NOLI, A. & FRANCO, L., 2009, “The ancient ports of Rome: new insights from engineers”, Archaeologia Maritima Mediterranea, 6.
[27] MURRAY, W., 1987, “Do modern winds equal ancient winds?”, Mediterranean Historical Review, 2, (p 139-167), https://doi.org/10.1080/09518968708569525.
[28] KEAY, S., 2014, “The Role Played by the Portus Augusti in Flows of Commerce between Rome and its Mediterranean Ports”, in “The Roman Economy”, edt. B. Woytek, Austrian Academy of Sciences, (147-192).
[29] Large merchantmen sailing from Alexandria would surely prefer calling at Puteoli than at Portus, as they would save some precious time to return back to Alexandria as soon as possible for their second yearly trip.